
Cross-posted from Nothing in Biology Makes Sense.
When evolutionary biologists think about sex, we often think of parasites, too. That’s not because we’re paranoid about sexually transmitted infections—though I’d like to think that biologists are more rigorous users of safer sex practices than the general population. It’s because coevolution with parasites is thought to be a major evolutionary reason for sexual reproduction.
This is the Red Queen hypothesis, named for the character in Lewis Carroll’s Through the Looking Glass who declares that “it takes all the running you can do to keep in the same place.” Parasite populations are constantly evolving new ways to infest and infect their hosts, the thinking goes. This means that a host individual who mixes her genes with another member of her species is more likely to give birth to offspring that carry new combinations of anti-parasite genes.
But although sex is the, er, sexiest prediction of the Red Queen, it’s not the whole story. What matters to the Red Queen is mixing up genetic material—and there’s more to that than the act of making the beast with two genomes. For instance, in the course of meiosis, the process by which sex cells are formed, chromosomes carrying different alleles for the same genes can “cross over,” breaking up and re-assembling new combinations of those genes. Recombination like this can re-mix the genes of species that reproduce mostly without sex; and the Red Queen implies that coevolution should favor higher rates of recombination even in sexual species.
That’s the case for the red flour beetle, the subject of a study just released online by the open-access journal BMC Evolutionary Biology. In an coevolutionary experiment that pits this worldwide household pest against deadly parasites, the authors show that parasites prompt higher rates of recombination in the beetles, just as the Red Queen predicts.
The red flour beetle, Tribolium castanaeum, is named for its predilection for stored grain products. This food preference makes the tiny beetles particularly easy to raise in the lab, where they’ve been useful enough as a study organism to rate a genome project, which was completed in 2008.
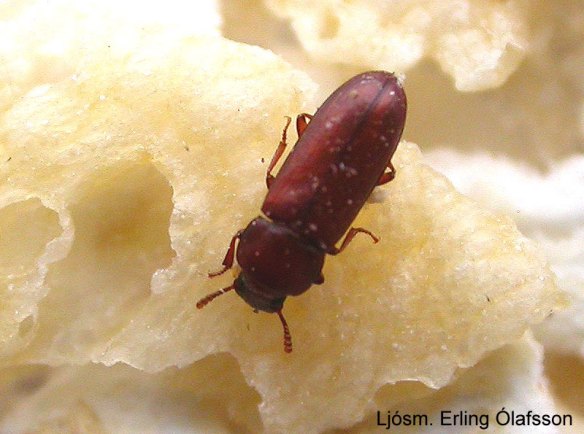
Tribolium castanaeum reproduces strictly sexually. But, like any other biological trait or process, the beetle’s rate of recombination can vary, and evolve. And, as I’ve explained above, the Red Queen suggests that selection by parasites should favor higher rates of recombination. So the authors of the new study set experimental populations of the beetle to evolve either in parasite-free habitats, or under attack by Nosema whitei, a protozoan that infects and kills flour beetle larvae.
The team started experimental populations of beetles (fed on organic flour, natch) in each of the two treatments with eight different genetic lines, maintaining them at a constant population by collecting 500 beetles at the end of each generation to start the next generation. To make the coevolution treatment coevolutionary, the authors also transferred spores of the parasite produced in the previous generation to infect each new generation of beetles.
After 11 generations of coevolution, the authors sampled male beetles from four of the experimental populations in each treatment, and mated them with females from the same genetic line. By collecting the genotypes of the sampled males for a small number of strategically chosen genes, and comparing them to the genotypes of the males’ offspring, it was then possible to identify recombination events—offspring who had combinations of alleles at different genes that weren’t seen in their fathers.
And, indeed, the frequency of recombination—the proportion of offspring whose genetics showed signs of recombination events when compared to their fathers—was greater in the experimental lines that coevolved with Nosema whitei.
That’s a fairly remarkable result for a simple, relatively short selection experiment, and to my knowledge it’s the first of its kind to deal with recombination, as opposed to sex. There are a few study systems in which natural populations show signs of coping with parasites by having more sex, including C.J.’s favorite mollusks, and there is one good experimental example in which the worm Caenorhabditis elegans evolved to reproduce sexually when confronted with bacterial parasites. But this study marks a new bit of empirical support for the Red Queen: coevolution acting to boost the gene-mixing benefits of sex. ◼
References
Kerstes, N., Berenos, C., Schmid-Hempel, P., & Wegner, K. (2012). Antagonistic experimental coevolution with a parasite increases host recombination frequency BMC Evolutionary Biology, 12 (1) DOI: 10.1186/1471-2148-12-18
Morran, L., Schmidt, O., Gelarden, I., Parrish, R., & Lively, C. (2011). Running with the Red Queen: Host-parasite coevolution selects for biparental sex. Science, 333 (6039), 216-218 DOI: 10.1126/science.1206360